Exoplanet
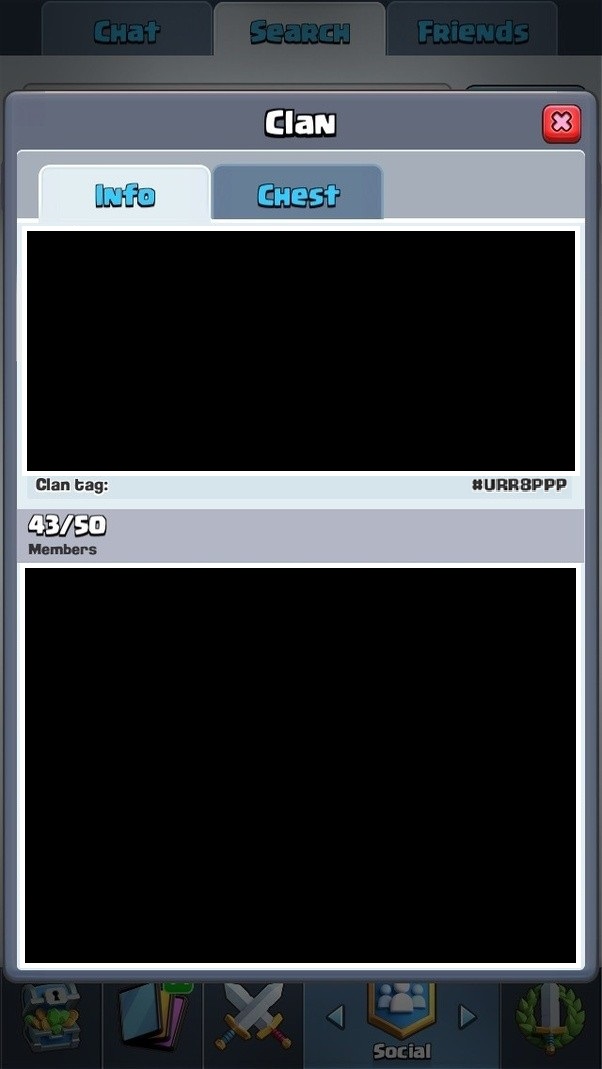

@media all and (max-width:720px).mw-parser-output .tmulti>.thumbinnerwidth:100%!important;max-width:none!important.mw-parser-output .tmulti .tsinglefloat:none!important;max-width:none!important;width:100%!important;text-align:center

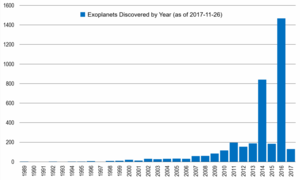


An exoplanet (UK: /ˈɛk.soʊˌplæn.ɪt/, US: /ˌɛk.soʊˈplæn.ɪt/)[4] or extrasolar planet is a planet outside our solar system. The first evidence of an exoplanet was noted as early as 1917, but was not recognized as such.[5] However, the first scientific detection of an exoplanet was in 1988, although it was not accepted as an exoplanet until later. The first confirmed detection occurred in 1992. As of 1 September 2018, there are 3,823 confirmed planets in 2,860 systems, with 632 systems having more than one planet.[6]
The High Accuracy Radial Velocity Planet Searcher (HARPS), since 2004) has discovered about a hundred exoplanets while the Kepler space telescope (since 2009) has found more than two thousand. Kepler has also detected a few thousand[7][8] candidate planets,[9][10] of which about 11% may be false positives.[11]
In several cases, multiple planets have been observed around a star.[12]
About 1 in 5 Sun-like stars[a] have an "Earth-sized"[b] planet in the habitable zone.[c] Assuming there are 200 billion stars in the Milky Way,[d] one can hypothesize that there are 11 billion potentially habitable Earth-sized planets in the Milky Way, rising to 40 billion if planets orbiting the numerous red dwarfs are included.[13]
The least massive planet known is Draugr (also known as PSR B1257+12 A or PSR B1257+12 b), which is about twice the mass of the Moon. The most massive planet listed on the NASA Exoplanet Archive is HR 2562 b,[14][15] about 30 times the mass of Jupiter, although according to some definitions of a planet, it is too massive to be a planet and may be a brown dwarf instead. There are planets that are so near to their star that they take only a few hours to orbit and there are others so far away that they take thousands of years to orbit. Some are so far out that it is difficult to tell whether they are gravitationally bound to the star. Almost all of the planets detected so far are within the Milky Way. Nonetheless, evidence suggests that extragalactic planets, exoplanets further away in galaxies beyond the local Milky Way galaxy, may exist.[16][17] The nearest exoplanet is Proxima Centauri b, located 4.2 light-years (1.3 parsecs) from Earth and orbiting Proxima Centauri, the closest star to the Sun.[18]
The discovery of exoplanets has intensified interest in the search for extraterrestrial life. There is special interest in planets that orbit in a star's habitable zone, where it is possible for liquid water, a prerequisite for life on Earth, to exist on the surface. The study of planetary habitability also considers a wide range of other factors in determining the suitability of a planet for hosting life.[19]
Besides exoplanets, there are also rogue planets, which do not orbit any star and which tend to be considered separately, especially if they are gas giants, in which case they are often counted, like WISE 0855−0714, as sub-brown dwarfs.[20] The rogue planets in the Milky Way possibly number in the billions (or more).[21][22]
Contents
1 Nomenclature
2 History of detection
2.1 Early speculations
2.2 Discredited claims
2.3 Confirmed discoveries
2.4 Candidate discoveries
3 Methodology
3.1 Detection techniques
4 Formation and evolution
5 Planet-hosting stars
6 General features
6.1 Color and brightness
6.2 Magnetic field
6.3 Plate tectonics
6.4 Volcanism
6.5 Rings
6.6 Moons
6.7 Atmospheres
6.8 Insolation pattern
7 See also
8 Notes
9 References
10 Further reading
11 External links
Nomenclature

Exoplanet HIP 65426b is the first discovered planet around star HIP 65426.[23]
The convention for designating exoplanets is an extension of the system used for designating multiple-star systems as adopted by the International Astronomical Union (IAU). For exoplanets orbiting a single star, the designation is normally formed by taking the name or, more commonly, designation of its parent star and adding a lower case letter.[24] The first planet discovered in a system is given the designation "b" (the parent star is considered to be "a") and later planets are given subsequent letters. If several planets in the same system are discovered at the same time, the closest one to the star gets the next letter, followed by the other planets in order of orbital size. A provisional IAU-sanctioned standard exists to accommodate the designation of circumbinary planets. A limited number of exoplanets have IAU-sanctioned proper names. Other naming systems exist.
History of detection
For centuries scientists, philosophers, and science fiction writers suspected that extrasolar planets existed,[25] but there was no way of detecting them or of knowing their frequency or how similar they might be to the planets of the Solar System. Various detection claims made in the nineteenth century were rejected by astronomers. The first evidence of an exoplanet was noted as early as 1917, but was not recognized as such.[5] The first suspected scientific detection of an exoplanet occurred in 1988. Shortly afterwards, the first confirmed detection came in 1992, with the discovery of several terrestrial-mass planets orbiting the pulsar PSR B1257+12.[26] The first confirmation of an exoplanet orbiting a main-sequence star was made in 1995, when a giant planet was found in a four-day orbit around the nearby star 51 Pegasi. Some exoplanets have been imaged directly by telescopes, but the vast majority have been detected through indirect methods, such as the transit method and the radial-velocity method. As of February 2018, researchers at the Chandra X-ray Observatory, combined with a planet detection technique called microlensing, found evidence that there are potentially one trillion extragalactic exoplanets, stating "Some of these exoplanets are as (relatively) small as the moon, while others are as massive as Jupiter. Unlike Earth, most of the exoplanets are not tightly bound to stars, so they're actually wandering through space or loosely orbiting between stars. We can estimate that the number of planets in this [faraway] galaxy is more than a trillion."[27]
Early speculations
“ | This space we declare to be infinite... In it are an infinity of worlds of the same kind as our own. | ” |
— Giordano Bruno (1584)[28] |
In the sixteenth century the Italian philosopher Giordano Bruno, an early supporter of the Copernican theory that Earth and other planets orbit the Sun (heliocentrism), put forward the view that the fixed stars are similar to the Sun and are likewise accompanied by planets.
In the eighteenth century the same possibility was mentioned by Isaac Newton in the "General Scholium" that concludes his Principia. Making a comparison to the Sun's planets, he wrote "And if the fixed stars are the centres of similar systems, they will all be constructed according to a similar design and subject to the dominion of One."[29]
In 1952, more than 40 years before the first hot Jupiter was discovered, Otto Struve wrote that there is no compelling reason why planets could not be much closer to their parent star than is the case in the Solar System, and proposed that Doppler spectroscopy and the transit method could detect super-Jupiters in short orbits.[30]
Discredited claims
Claims of exoplanet detections have been made since the nineteenth century. Some of the earliest involve the binary star 70 Ophiuchi. In 1855 William Stephen Jacob at the East India Company's Madras Observatory reported that orbital anomalies made it "highly probable" that there was a "planetary body" in this system.[31] In the 1890s, Thomas J. J. See of the University of Chicago and the United States Naval Observatory stated that the orbital anomalies proved the existence of a dark body in the 70 Ophiuchi system with a 36-year period around one of the stars.[32] However, Forest Ray Moulton published a paper proving that a three-body system with those orbital parameters would be highly unstable.[33] During the 1950s and 1960s, Peter van de Kamp of Swarthmore College made another prominent series of detection claims, this time for planets orbiting Barnard's Star.[34] Astronomers now generally regard all the early reports of detection as erroneous.[35]
In 1991 Andrew Lyne, M. Bailes and S. L. Shemar claimed to have discovered a pulsar planet in orbit around PSR 1829-10, using pulsar timing variations.[36] The claim briefly received intense attention, but Lyne and his team soon retracted it.[37]
Confirmed discoveries

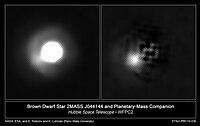
2MASS J044144 is a brown dwarf with a companion about 5–10 times the mass of Jupiter. It is not clear whether this companion object is a sub-brown dwarf or a planet.
As of 1 September 2018, a total of 3,823 confirmed exoplanets are listed in the Extrasolar Planets Encyclopaedia, including a few that were confirmations of controversial claims from the late 1980s.[6] The first published discovery to receive subsequent confirmation was made in 1988 by the Canadian astronomers Bruce Campbell, G. A. H. Walker, and Stephenson Yang of the University of Victoria and the University of British Columbia.[38] Although they were cautious about claiming a planetary detection, their radial-velocity observations suggested that a planet orbits the star Gamma Cephei. Partly because the observations were at the very limits of instrumental capabilities at the time, astronomers remained skeptical for several years about this and other similar observations. It was thought some of the apparent planets might instead have been brown dwarfs, objects intermediate in mass between planets and stars. In 1990 additional observations were published that supported the existence of the planet orbiting Gamma Cephei,[39] but subsequent work in 1992 again raised serious doubts.[40] Finally, in 2003, improved techniques allowed the planet's existence to be confirmed.[41]

Coronagraphic image of AB Pictoris showing a companion (bottom left), which is either a brown dwarf or a massive planet. The data was obtained on 16 March 2003 with NACO on the VLT, using a 1.4 arcsec occulting mask on top of AB Pictoris.
On 9 January 1992, radio astronomers Aleksander Wolszczan and Dale Frail announced the discovery of two planets orbiting the pulsar PSR 1257+12.[26] This discovery was confirmed, and is generally considered to be the first definitive detection of exoplanets. Follow-up observations solidified these results, and confirmation of a third planet in 1994 revived the topic in the popular press.[42] These pulsar planets are thought to have formed from the unusual remnants of the supernova that produced the pulsar, in a second round of planet formation, or else to be the remaining rocky cores of gas giants that somehow survived the supernova and then decayed into their current orbits.
On 6 October 1995, Michel Mayor and Didier Queloz of the University of Geneva announced the first definitive detection of an exoplanet orbiting a main-sequence star, namely the nearby G-type star 51 Pegasi.[43][44] This discovery, made at the Observatoire de Haute-Provence, ushered in the modern era of exoplanetary discovery. Technological advances, most notably in high-resolution spectroscopy, led to the rapid detection of many new exoplanets: astronomers could detect exoplanets indirectly by measuring their gravitational influence on the motion of their host stars. More extrasolar planets were later detected by observing the variation in a star's apparent luminosity as an orbiting planet passed in front of it.
Initially, most known exoplanets were massive planets that orbited very close to their parent stars. Astronomers were surprised by these "hot Jupiters", because theories of planetary formation had indicated that giant planets should only form at large distances from stars. But eventually more planets of other sorts were found, and it is now clear that hot Jupiters make up the minority of exoplanets. In 1999, Upsilon Andromedae became the first main-sequence star known to have multiple planets.[45]Kepler-16 contains the first discovered planet that orbits around a binary main-sequence star system.[46]
On 26 February 2014, NASA announced the discovery of 715 newly verified exoplanets around 305 stars by the Kepler Space Telescope. These exoplanets were checked using a statistical technique called "verification by multiplicity".[47][48][49] Prior to these results, most confirmed planets were gas giants comparable in size to Jupiter or larger as they are more easily detected, but the Kepler planets are mostly between the size of Neptune and the size of Earth.[47]
On 23 July 2015, NASA announced Kepler-452b, a near-Earth-size planet orbiting the habitable zone of a G2-type star.[50]
Candidate discoveries
As of June 2017, NASA's Kepler mission had identified more than 5,000 planetary candidates,[51] several of them being nearly Earth-sized and located in the habitable zone, some around Sun-like stars.[7][8][52]


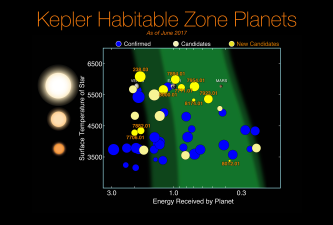
Methodology
The first exoplanet was detected on 6 October 1995, and was named 51 Pegasi b.[55] When an extrasolar planet is observed to transit its parent star, astronomers are able to assess some physical properties of the planet from an interstellar distance, including planetary mass and size, which in turn provide fundamental constraints on models of their physical structure.[56] Furthermore, such events afford the opportunity to study the dynamics and chemistry of its atmosphere.[56]
Statistical surveys and individual characterization are the keys to addressing the fundamental questions in exoplanetology.[57] As of August 2016, varying techniques have been used to discover 3,502 exoplanets.[58] Documenting the properties of a large sample exoplanets at various ages, orbiting their parent stars of various types, will contribute to increased understanding —or better models— of planetary formation (accretion), geological evolution, orbit migration,[57][59] and their potential habitability.[60] Characterizing the atmospheres of extrasolar planets is the new frontier in exoplanetary science.[61]
Detection techniques

Measuring the flow of gas within a protoplanetary disc allows the detection of exoplanets.[62]
About 97% of all the confirmed exoplanets have been discovered by indirect techniques of detection, mainly by radial velocity measurements and transit monitoring techniques.[60] The following methods have proved successful for discovering a new planet or confirming an already discovered planet:[63]
- Radial velocity
- Gravitational microlensing
- Direct imaging
- Polarimetry
- Astrometry
- Transit photometry
- Reflection/emission modulations
- Light variations due to relativistic beaming
- Light variations due to ellipsoidal variations
- Timing variations
- Pulsar timing
- Variable star timing
- Transit timing variation method
- Transit duration variation method
- Eclipsing binary minima timing
Formation and evolution
Planets form within a few tens of millions of years of their star forming.[64][65][66] The planets of the Solar System can only be observed in their current state, but observations of different planetary systems of varying ages allows us to observe planets at different stages of evolution. Available observations range from young proto-planetary disks where planets are still forming [67] to planetary systems of over 10 Gyr old.[68] When terrestrial planets form in a gaseous protoplanetary disk,[69] they have hydrogen envelopes that cool and contract over time and, depending on the mass of the planet, some or all of the hydrogen is eventually lost to space. This means that even terrestrial planets may start off with large radii if they form early enough.[70][71][72] An example is Kepler-51b which has only about twice the mass of Earth but is almost the size of Saturn which is a hundred times the mass of Earth. Kepler-51b is quite young at a few hundred million years old.[73]
Planet-hosting stars

The Morgan-Keenan spectral classification

Artist’s impression of exoplanet orbiting two stars.[74]
There is at least one planet on average per star.[12]
About 1 in 5 Sun-like stars[a] have an "Earth-sized"[b] planet in the habitable zone.[75]
Most known exoplanets orbit stars roughly similar to the Sun, i.e. main-sequence stars of spectral categories F, G, or K. Lower-mass stars (red dwarfs, of spectral category M) are less likely to have planets massive enough to be detected by the radial-velocity method.[76][77] Despite this, several tens of planets around red dwarfs have been discovered by the Kepler spacecraft, which uses the transit method to detect smaller planets.
Using data from Kepler, a correlation has been found between the metallicity of a star and the probability that the star host planets. Stars with higher metallicity are more likely to have planets, especially giant planets, than stars with lower metallicity.[78]
Some planets orbit one member of a binary star system,[79] and several circumbinary planets have been discovered which orbit around both members of binary star. A few planets in triple star systems are known[80] and one in the quadruple system Kepler-64.
General features
Color and brightness

This color–color diagram compares the colors of planets in the Solar System to exoplanet HD 189733b. The exoplanet's deep blue color is produced by silicate droplets, which scatter blue light in its atmosphere.
In 2013 the color of an exoplanet was determined for the first time. The best-fit albedo measurements of HD 189733b suggest that it is deep dark blue.[81][82] Later that same year, the colors of several other exoplanets were determined, including GJ 504 b which visually has a magenta color,[83] and Kappa Andromedae b, which if seen up close would appear reddish in color.[84]
The apparent brightness (apparent magnitude) of a planet depends on how far away the observer is, how reflective the planet is (albedo), and how much light the planet receives from its star, which depends on how far the planet is from the star and how bright the star is. So, a planet with a low albedo that is close to its star can appear brighter than a planet with high albedo that is far from the star.[85]
The darkest known planet in terms of geometric albedo is TrES-2b, a hot Jupiter that reflects less than 1% of the light from its star, making it less reflective than coal or black acrylic paint. Hot Jupiters are expected to be quite dark due to sodium and potassium in their atmospheres but it is not known why TrES-2b is so dark—it could be due to an unknown chemical compound.[86][87][88]
For gas giants, geometric albedo generally decreases with increasing metallicity or atmospheric temperature unless there are clouds to modify this effect. Increased cloud-column depth increases the albedo at optical wavelengths, but decreases it at some infrared wavelengths. Optical albedo increases with age, because older planets have higher cloud-column depths. Optical albedo decreases with increasing mass, because higher-mass giant planets have higher surface gravities, which produces lower cloud-column depths. Also, elliptical orbits can cause major fluctuations in atmospheric composition, which can have a significant effect.[89]
There is more thermal emission than reflection at some near-infrared wavelengths for massive and/or young gas giants. So, although optical brightness is fully phase-dependent, this is not always the case in the near infrared.[89]
Temperatures of gas giants reduce over time and with distance from their star. Lowering the temperature increases optical albedo even without clouds. At a sufficiently low temperature, water clouds form, which further increase optical albedo. At even lower temperatures ammonia clouds form, resulting in the highest albedos at most optical and near-infrared wavelengths.[89]
Magnetic field
In 2014, a magnetic field around HD 209458 b was inferred from the way hydrogen was evaporating from the planet. It is the first (indirect) detection of a magnetic field on an exoplanet. The magnetic field is estimated to be about one tenth as strong as Jupiter's.[90][91]
Interaction between a close-in planet's magnetic field and a star can produce spots on the star in a similar way to how the Galilean moons produce aurorae on Jupiter.[92]Auroral radio emissions could be detected with radio telescopes such as LOFAR.[93][94]
The radio emissions could enable determination of the rotation rate of a planet which is difficult to detect otherwise.[95]
Earth's magnetic field results from its flowing liquid metallic core, but in massive super-Earths with high pressure, different compounds may form which do not match those created under terrestrial conditions. Compounds may form with greater viscosities and high melting temperatures which could prevent the interiors from separating into different layers and so result in undifferentiated coreless mantles. Forms of magnesium oxide such as MgSi3O12 could be a liquid metal at the pressures and temperatures found in super-Earths and could generate a magnetic field in the mantles of super-Earths.[96][97]
Hot Jupiters have been observed to have a larger radius than expected. This could be caused by the interaction between the stellar wind and the planet's magnetosphere creating an electric current through the planet that heats it up causing it to expand. The more magnetically active a star is the greater the stellar wind and the larger the electric current leading to more heating and expansion of the planet. This theory matches the observation that stellar activity is correlated with inflated planetary radii.[98]
In August 2018, scientists announced the transformation of gaseous deuterium into a liquid metallic form. This may help researchers better understand giant gas planets, such as Jupiter, Saturn and related exoplanets, since such planets are thought to contain a lot of liquid metallic hydrogen, which may be responsible for their observed powerful magnetic fields.[99][100]
Plate tectonics
In 2007, two independent teams of researchers came to opposing conclusions about the likelihood of plate tectonics on larger super-Earths[101][102] with one team saying that plate tectonics would be episodic or stagnant[103] and the other team saying that plate tectonics is very likely on super-Earths even if the planet is dry.[104]
If super-Earths have more than 80 times as much water as Earth then they become ocean planets with all land completely submerged. However, if there is less water than this limit, then the deep water cycle will move enough water between the oceans and mantle to allow continents to exist.[105][106]
Volcanism
Large surface temperature variations on 55 Cancri e have been attributed to possible volcanic activity releasing large clouds of dust which blanket the planet and block thermal emissions.[107][108]
Rings
The star 1SWASP J140747.93-394542.6 is orbited by an object that is circled by a ring system much larger than Saturn's rings. However, the mass of the object is not known; it could be a brown dwarf or low-mass star instead of a planet.[109][110]
The brightness of optical images of Fomalhaut b could be due to starlight reflecting off a circumplanetary ring system with a radius between 20 and 40 times that of Jupiter's radius, about the size of the orbits of the Galilean moons.[111]
The rings of the Solar System's gas giants are aligned with their planet's equator. However, for exoplanets that orbit close to their star, tidal forces from the star would lead to the outermost rings of a planet being aligned with the planet's orbital plane around the star. A planet's innermost rings would still be aligned with the planet's equator so that if the planet has a tilted rotational axis, then the different alignments between the inner and outer rings would create a warped ring system.[112]
Moons
In December 2013 a candidate exomoon of a rogue planet was announced.[113] No exomoons have been confirmed so far.
Atmospheres
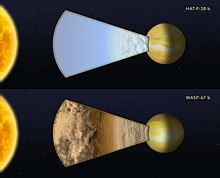
Clear versus cloudy atmospheres on two exoplanets.[114]
Atmospheres have been detected around several exoplanets. The first to be observed was HD 209458 b in 2001.[115]
KIC 12557548 b is a small rocky planet, very close to its star, that is evaporating and leaving a trailing tail of cloud and dust like a comet.[116] The dust could be ash erupting from volcanos and escaping due to the small planet's low surface-gravity, or it could be from metals that are vaporized by the high temperatures of being so close to the star with the metal vapor then condensing into dust.[117]
In June 2015, scientists reported that the atmosphere of GJ 436 b was evaporating, resulting in a giant cloud around the planet and, due to radiation from the host star, a long trailing tail 14×10^6 km (9×10^6 mi) long.[118]
In May 2017, glints of light from Earth, seen as twinkling from an orbiting satellite a million miles away, were found to be reflected light from ice crystals in the atmosphere.[119][120] The technology used to determine this may be useful in studying the atmospheres of distant worlds, including those of exoplanets.
Insolation pattern
Tidally locked planets in a 1:1 spin–orbit resonance would have their star always shining directly overhead on one spot which would be hot with the opposite hemisphere receiving no light and being freezing cold. Such a planet could resemble an eyeball with the hotspot being the pupil.[121] Planets with an eccentric orbit could be locked in other resonances. 3:2 and 5:2 resonances would result in a double-eyeball pattern with hotspots in both eastern and western hemispheres.[122] Planets with both an eccentric orbit and a tilted axis of rotation would have more complicated insolation patterns.[123]
As more planets are discovered, the field of exoplanetology continues to grow into a deeper study of extrasolar worlds, and will ultimately tackle the prospect of life on planets beyond the Solar System.[60] At cosmic distances, life
can only be detected if it is developed at a planetary scale and strongly modified the planetary
environment, in such a way that the modifications cannot be explained by classical physico-chemical processes (out of equilibrium processes).[60] For example, molecular oxygen (O
2) in the atmosphere of Earth is a result of photosynthesis by living plants and many kinds of microorganisms, so it can be used as an indication of life on exoplanets, although small amounts of oxygen could also be produced by non-biological means.[124] Furthermore, a potentially habitable planet must orbit a stable star at a distance within which planetary-mass objects with sufficient atmospheric pressure can support liquid water at their surfaces.[125][126]
See also
- Exocomet
- Exomoon
- Exoplanetology
- Extragalactic planet
- List of exoplanets
- List of exoplanet extremes
- List of exoplanet search projects
- List of planets
- Nexus for Exoplanet System Science
- Planetary system
- Ultra-short period planet (USP)
Notes
^ ab For the purpose of this 1 in 5 statistic, "Sun-like" means G-type star. Data for Sun-like stars was not available so this statistic is an extrapolation from data about K-type stars
^ ab For the purpose of this 1 in 5 statistic, Earth-sized means 1–2 Earth radii
^ For the purpose of this 1 in 5 statistic, "habitable zone" means the region with 0.25 to 4 times Earth's stellar flux (corresponding to 0.5–2 AU for the Sun).
^ About 1/4 of stars are GK Sun-like stars. The number of stars in the galaxy is not accurately known, but assuming 200 billion stars in total, the Milky Way would have about 50 billion Sun-like (GK) stars, of which about 1 in 5 (22%) or 11 billion would be Earth-sized in the habitable zone. Including red dwarfs would increase this to 40 billion.
References
^ "Planet Population is Plentiful". ESO. 11 January 2012. Retrieved 13 January 2012.
^ Histogram Plots. Exoplanet.eu
^ "Exoplanet Transit Database: TrES-3b". astro.cz. Czech Astronomical Society. Retrieved 7 July 2015.
^ "exoplanet Meaning in the Cambridge English Dictionary".
^ ab Landau, Elizabeth (12 November 2017). "Overlooked Treasure: The First Evidence of Exoplanets". NASA. Retrieved 1 November 2017.
^ ab Schneider, J. "Interactive Extra-solar Planets Catalog". The Extrasolar Planets Encyclopedia. Retrieved 1 September 2018.
^ ab Jerry Colen (4 November 2013). "Kepler". nasa.gov. NASA. Archived from the original on 5 November 2013. Retrieved 4 November 2013.
^ ab Harrington, J. D.; Johnson, M. (4 November 2013). "NASA Kepler Results Usher in a New Era of Astronomy".
^ Tenenbaum, P.; Jenkins, J. M.; Seader, S.; Burke, C. J.; Christiansen, J. L.; Rowe, J. F.; Caldwell, D. A.; Clarke, B. D.; Li, J.; Quintana, E. V.; Smith, J. C.; Thompson, S. E.; Twicken, J. D.; Borucki, W. J.; Batalha, N. M.; Cote, M. T.; Haas, M. R.; Hunter, R. C.; Sanderfer, D. T.; Girouard, F. R.; Hall, J. R.; Ibrahim, K.; Klaus, T. C.; McCauliff, S. D.; Middour, C. K.; Sabale, A.; Uddin, A. K.; Wohler, B.; Barclay, T.; Still, M. (2013). "Detection of Potential Transit Signals in the First 12 Quarters of Kepler Mission Data". The Astrophysical Journal Supplement Series. 206: 5. arXiv:1212.2915. Bibcode:2013ApJS..206....5T. doi:10.1088/0067-0049/206/1/5.
^ "My God, it's full of planets! They should have sent a poet" (Press release). Planetary Habitability Laboratory, University of Puerto Rico at Arecibo. 3 January 2012.
^ Santerne, A.; Díaz, R. F.; Almenara, J.-M.; Lethuillier, A.; Deleuil, M.; Moutou, C. (2013). "Astrophysical false positives in exoplanet transit surveys: Why do we need bright stars?". SF2A-2013: Proceedings of the Annual meeting of the French Society of Astronomy and Astrophysics. Eds.: L. Cambresy: 555. arXiv:1310.2133[astro-ph.EP]. Bibcode:2013sf2a.conf..555S.
^ ab Cassan, A.; Kubas, D.; Beaulieu, J. -P.; Dominik, M.; Horne, K.; Greenhill, J.; Wambsganss, J.; Menzies, J.; Williams, A.; Jørgensen, U. G.; Udalski, A.; Bennett, D. P.; Albrow, M. D.; Batista, V.; Brillant, S.; Caldwell, J. A. R.; Cole, A.; Coutures, C.; Cook, K. H.; Dieters, S.; Prester, D. D.; Donatowicz, J.; Fouqué, P.; Hill, K.; Kains, N.; Kane, S.; Marquette, J. -B.; Martin, R.; Pollard, K. R.; Sahu, K. C. (11 January 2012). "One or more bound planets per Milky Way star from microlensing observations". Nature. 481 (7380): 167–169. arXiv:1202.0903. Bibcode:2012Natur.481..167C. doi:10.1038/nature10684. PMID 22237108.
^ Khan, Amina (4 November 2013). "Milky Way may host billions of Earth-size planets". Los Angeles Times. Retrieved 5 November 2013.
^ "HR 2562 b". Caltech. Retrieved 15 February 2018.
^ Konopacky, Quinn M.; Rameau, Julien; Duchêne, Gaspard; Filippazzo, Joseph C.; Giorla Godfrey, Paige A.; Marois, Christian; Nielsen, Eric L. (20 September 2016). "Discovery of a Substellar Companion to the Nearby Debris Disk Host HR 2562" (Full text). The Astrophysical Journal Letters. 829: 10. arXiv:1608.06660. Bibcode:2016ApJ...829L...4K. doi:10.3847/2041-8205/829/1/L4.
^ Zachos, Elaine (5 February 2018). "More Than a Trillion Planets Could Exist Beyond Our Galaxy – A new study gives the first evidence that exoplanets exist beyond the Milky Way". National Geographic Society. Retrieved 5 February 2018.
^ Mandelbaum, Ryan F. (5 February 2018). "Scientists Find Evidence of Thousands of Planets in Distant Galaxy". Gizmodo. Retrieved 5 February 2018.
^ Anglada-Escudé, Guillem; Amado, Pedro J.; Barnes, John; Berdiñas, Zaira M.; Butler, R. Paul; Coleman, Gavin A. L.; de la Cueva, Ignacio; Dreizler, Stefan; Endl, Michael (2016-08-25). "A terrestrial planet candidate in a temperate orbit around Proxima Centauri". Nature. 536 (7617): 437–440. arXiv:1609.03449. Bibcode:2016Natur.536..437A. doi:10.1038/nature19106. ISSN 0028-0836. PMID 27558064.
^ Overbye, Dennis (6 January 2015). "As Ranks of Goldilocks Planets Grow, Astronomers Consider What's Next". The New York Times.
^ Beichman, C.; Gelino, Christopher R.; Kirkpatrick, J. Davy; Cushing, Michael C.; Dodson-Robinson, Sally; Marley, Mark S.; Morley, Caroline V.; Wright, E. L. (2014). "WISE Y Dwarfs As Probes of the Brown Dwarf-Exoplanet Connection". The Astrophysical Journal. 783 (2): 68. arXiv:1401.1194. Bibcode:2014ApJ...783...68B. doi:10.1088/0004-637X/783/2/68.
^ Neil DeGrasse Tyson in Cosmos: A Spacetime Odyssey as referred to by National Geographic
^ Strigari, L. E.; Barnabè, M.; Marshall, P. J.; Blandford, R. D. (2012). "Nomads of the Galaxy". Monthly Notices of the Royal Astronomical Society. 423 (2): 1856–1865. arXiv:1201.2687. Bibcode:2012MNRAS.423.1856S. doi:10.1111/j.1365-2966.2012.21009.x. estimates 700 objects >10−6 solar masses (roughly the mass of Mars) per main-sequence star between 0.08 and 1 Solar mass, of which there are billions in the Milky Way.
^ "ESO's SPHERE Unveils its First Exoplanet". www.eso.org. Retrieved 7 July 2017.
^ "International Astronomical Union | IAU". www.iau.org. Retrieved 2017-01-29.
^ "1992 --"The Year the Milky Way's Planets Came to Life"". Daily Galaxy. 9 January 2017. Retrieved 15 January 2017.
^ ab Wolszczan, A.; Frail, D. A. (1992). "A planetary system around the millisecond pulsar PSR1257 + 12". Nature. 355 (6356): 145–147. Bibcode:1992Natur.355..145W. doi:10.1038/355145a0.
^ "These May Be the First Planets Found Outside Our Galaxy". National Geographic. 2018-02-05. Retrieved 2018-02-08.
^ Eli Maor (1987). "Chapter 24: The New Cosmology". To Infinity and Beyond: A Cultural History of the Infinite. Originally in De l'infinito universo et mondi [On the Infinite Universe and Worlds] by Giordano Bruno (1584). Boston, MA: Birkhäuser. p. 198. ISBN 978-1-4612-5396-9. Retrieved 9 July 2016.
^ Newton, Isaac; I. Bernard Cohen; Anne Whitman (1999) [1713]. The Principia: A New Translation and Guide. University of California Press. p. 940. ISBN 978-0-520-08816-0.
^ Struve, Otto (1952). "Proposal for a project of high-precision stellar radial velocity work". The Observatory. 72: 199–200. Bibcode:1952Obs....72..199S.
^ Jacob, W. S. (1855). "On Certain Anomalies presented by the Binary Star 70 Ophiuchi". Monthly Notices of the Royal Astronomical Society. 15 (9): 228–230. Bibcode:1855MNRAS..15..228J. doi:10.1093/mnras/15.9.228.
^ See, T. J. J. (1896). "Researches on the orbit of 70 Ophiuchi, and on a periodic perturbation in the motion of the system arising from the action of an unseen body". The Astronomical Journal. 16: 17–23. Bibcode:1896AJ.....16...17S. doi:10.1086/102368.
^ Sherrill, T. J. (1999). "A Career of Controversy: The Anomaly of T. J. J. See" (PDF). Journal for the History of Astronomy. 30 (98): 25–50. Bibcode:1999JHA....30...25S. doi:10.1177/002182869903000102.
^ van de Kamp, P. (1969). "Alternate dynamical analysis of Barnard's star". Astronomical Journal. 74: 757–759. Bibcode:1969AJ.....74..757V. doi:10.1086/110852.
^ Boss, Alan (2009). The Crowded Universe: The Search for Living Planets. Basic Books. pp. 31–32. ISBN 978-0-465-00936-7.
^ Bailes, M.; Lyne, A. G.; Shemar, S. L. (1991). "A planet orbiting the neutron star PSR1829–10". Nature. 352 (6333): 311–313. Bibcode:1991Natur.352..311B. doi:10.1038/352311a0.
^ Lyne, A. G.; Bailes, M. (1992). "No planet orbiting PS R1829–10". Nature. 355 (6357): 213. Bibcode:1992Natur.355..213L. doi:10.1038/355213b0.
^ Campbell, B.; Walker, G. A. H.; Yang, S. (1988). "A search for substellar companions to solar-type stars". The Astrophysical Journal. 331: 902. Bibcode:1988ApJ...331..902C. doi:10.1086/166608.
^ Lawton, A. T.; Wright, P. (1989). "A planetary system for Gamma Cephei?". Journal of the British Interplanetary Society. 42: 335–336. Bibcode:1989JBIS...42..335L.
^ Walker, G. A. H; Bohlender, D. A.; Walker, A. R.; Irwin, A. W.; Yang, S. L. S.; Larson, A. (1992). "Gamma Cephei – Rotation or planetary companion?". Astrophysical Journal Letters. 396 (2): L91–L94. Bibcode:1992ApJ...396L..91W. doi:10.1086/186524.
^ Hatzes, A. P.; Cochran, William D.; Endl, Michael; McArthur, Barbara; Paulson, Diane B.; Walker, Gordon A. H.; Campbell, Bruce; Yang, Stephenson (2003). "A Planetary Companion to Gamma Cephei A". Astrophysical Journal. 599 (2): 1383–1394. arXiv:astro-ph/0305110. Bibcode:2003ApJ...599.1383H. doi:10.1086/379281.
^ Holtz, Robert (22 April 1994). "Scientists Uncover Evidence of New Planets Orbiting Star". Los Angeles Times via The Tech Online.
^ Mayor, M.; Queloz, D. (1995). "A Jupiter-mass companion to a solar-type star". Nature. 378 (6555): 355–359. Bibcode:1995Natur.378..355M. doi:10.1038/378355a0.
^ Gibney, Elizabeth (18 December 2013). "In search of sister earths". Nature. 504 (7480): 361. Bibcode:2013Natur.504..357.. doi:10.1038/504357a.
^ Lissauer, J. J. (1999). "Three planets for Upsilon Andromedae". Nature. 398 (6729): 659. Bibcode:1999Natur.398..659L. doi:10.1038/19409.
^ Doyle, L. R.; Carter, J. A.; Fabrycky, D. C.; Slawson, R. W.; Howell, S. B.; Winn, J. N.; Orosz, J. A.; Prša, A.; Welsh, W. F.; Quinn, S. N.; Latham, D.; Torres, G.; Buchhave, L. A.; Marcy, G. W.; Fortney, J. J.; Shporer, A.; Ford, E. B.; Lissauer, J. J.; Ragozzine, D.; Rucker, M.; Batalha, N.; Jenkins, J. M.; Borucki, W. J.; Koch, D.; Middour, C. K.; Hall, J. R.; McCauliff, S.; Fanelli, M. N.; Quintana, E. V.; Holman, M. J.; et al. (2011). "Kepler-16: A Transiting Circumbinary Planet". Science. 333 (6049): 1602–6. arXiv:1109.3432. Bibcode:2011Sci...333.1602D. doi:10.1126/science.1210923. PMID 21921192.
^ ab Johnson, Michele; Harrington, J.D. (26 February 2014). "NASA's Kepler Mission Announces a Planet Bonanza, 715 New Worlds". NASA. Retrieved 26 February 2014.
^ Wall, Mike (26 February 2014). "Population of Known Alien Planets Nearly Doubles as NASA Discovers 715 New Worlds". space.com. Retrieved 27 February 2014.
^ Jonathan Amos (26 February 2014). "Kepler telescope bags huge haul of planets". BBC News. Retrieved 27 February 2014.
^ Johnson, Michelle; Chou, Felicia (23 July 2015). "NASA's Kepler Mission Discovers Bigger, Older Cousin to Earth". NASA.
^ Johnson, Michele (9 June 2017). "Media Invited to NASA's Kepler Science Conference". NASA. Retrieved 20 June 2017.
^ "NASA's Exoplanet Archive KOI table". NASA. Retrieved 28 February 2014.
^ Lewin, Sarah (19 June 2017). "NASA's Kepler Space Telescope Finds Hundreds of New Exoplanets, Boosts Total to 4,034". NASA. Retrieved 19 June 2017.
^ Overbye, Dennis (19 June 2017). "Earth-Size Planets Among Final Tally of NASA's Kepler Telescope". The New York Times.
^ Exoplanet Anniversary: From Zero to Thousands in 20 Years. NASA News, 6 October 2015.
^ ab Charbonneau, David (May 2008). "The Era of Comparative Exoplanetology." American Astronomical Society. AAS Meeting #212, #54.01; Bulletin of the American Astronomical Society, Vol. 40, p. 250
^ ab Desert, Jean-Michel; Deming, Drake; Knutson, Heather; Bean, Jacob; Fortney, Jonathan; Burrows, Adam; Showman, Adam. "New Frontiers for Comparative Exoplanetology In the Era of Kepler". Spitzer Proposal ID 90092. Bibcode:2012sptz.prop90092D. CS1 maint: Uses authors parameter (link)
^
"The Extrasolar Planets Encyclopaedia". Retrieved 2016-08-18.
^ Kraus, Adam L.; Ireland, Michael J. (27 December 2012). "LkCa 15: A YOUNG EXOPLANET CAUGHT AT FORMATION?". The Astrophysical Journal. 745 (1): 5. arXiv:1110.3808. Bibcode:2012ApJ...745....5K. doi:10.1088/0004-637X/745/1/5.
^ abcd Ollivier, Marc; Maurel, Marie-Christine (2014). "Planetary Environments and Origins of Life: How to reinvent the study of Origins of Life on the Earth and Life in the" (PDF). BIO Web of Conferences 2. 2: 00001. doi:10.1051/bioconf/20140200001. Retrieved 2015-09-11.
^ Madhusudhan, Nikku; Agúndez, Marcelino; Moses, Julianne I.; Hu, Yongyun (20 Apr 2016). "Exoplanetary Atmospheres – Chemistry, Formation Conditions, and Habitability". Space Science Reviews. 205 (1): 285–348. arXiv:1604.06092. Bibcode:2016SSRv..205..285M. doi:10.1007/s11214-016-0254-3. PMC 5207327
. PMID 28057962.
^ "ALMA Discovers Trio of Infant Planets around Newborn Star – Novel technique to find youngest planets in our galaxy". www.eso.org. Retrieved 15 June 2018.
^ Ollivier M., Encrenaz T., Roques F., Selsis F., Casoli F., Planetary Systems – Detection, Formation and Habitability of Extrasolar Planets, Springer, Berlin (2008)
^ Mamajek, Eric E.; Usuda, Tomonori; Tamura, Motohide; Ishii, Miki (2009). "Initial Conditions of Planet Formation: Lifetimes of Primordial Disks". AIP Conference Proceedings. Exoplanets and Disks: Their Formation and Diversity: Proceedings of the International Conference. 1158. p. 3. arXiv:0906.5011. Bibcode:2009AIPC.1158....3M. doi:10.1063/1.3215910.
^ Rice, W. K. M.; Armitage, P. J. (2003). "On the Formation Timescale and Core Masses of Gas Giant Planets". The Astrophysical Journal. 598: L55–L58. arXiv:astro-ph/0310191. Bibcode:2003ApJ...598L..55R. doi:10.1086/380390.
^ Yin, Q.; Jacobsen, S. B.; Yamashita, K.; Blichert-Toft, J.; Télouk, P.; Albarède, F. (2002). "A short timescale for terrestrial planet formation from Hf–W chronometry of meteorites". Nature. 418 (6901): 949–952. Bibcode:2002Natur.418..949Y. doi:10.1038/nature00995. PMID 12198540.
^ Calvet, Nuria; D'Alessio, Paola; Hartmann, Lee; Wilner, David; Walsh, Andrew; Sitko, Michael (2001). "Evidence for a developing gap in a 10 Myr old protoplanetary disk". The Astrophysical Journal. 568 (2): 1008–1016. arXiv:astro-ph/0201425. Bibcode:2002ApJ...568.1008C. doi:10.1086/339061.
^ Fridlund, Malcolm; Gaidos, Eric; Barragán, Oscar; Persson, Carina; Gandolfi, Davide; Cabrera, Juan; Hirano, Teruyuki; Kuzuhara, Masayuki; Csizmadia, Sz; Nowak, Grzegorz; Endl, Michael; Grziwa, Sascha; Korth, Judith; Pfaff, Jeremias; Bitsch, Bertram; Johansen, Anders; Mustill, Alexander; Davies, Melvyn; Deeg, Hans; Palle, Enric; Cochran, William; Eigmüller, Philipp; Erikson, Anders; Guenther, Eike; Hatzes, Artie; Kiilerich, Amanda; Kudo, Tomoyuki; MacQueen, Philipp; Narita, Norio; Nespral, David; Pätzold, Martin; Prieto-Arranz, Jorge; Rauer, Heike; van Eylen, Vincent (28 April 2017). "EPIC210894022b −A short period super-Earth transiting a metal poor, evolved old star". Astronomy & Astrophysics. arXiv:1704.08284.
^ D'Angelo, G.; Durisen, R. H.; Lissauer, J. J. (2011). "Giant Planet Formation". In S. Seager. Exoplanets. University of Arizona Press, Tucson, AZ. pp. 319–346. arXiv:1006.5486. Bibcode:2010exop.book..319D.
^ Lammer, H.; Stokl, A.; Erkaev, N. V.; Dorfi, E. A.; Odert, P.; Gudel, M.; Kulikov, Y. N.; Kislyakova, K. G.; Leitzinger, M. (2014). "Origin and loss of nebula-captured hydrogen envelopes from 'sub'- to 'super-Earths' in the habitable zone of Sun-like stars". Monthly Notices of the Royal Astronomical Society. 439 (4): 3225–3238. arXiv:1401.2765. Bibcode:2014MNRAS.439.3225L. doi:10.1093/mnras/stu085.
^ Johnson, R. E. (2010). "Thermally-Diven Atmospheric Escape". The Astrophysical Journal. 716 (2): 1573–1578. arXiv:1001.0917. Bibcode:2010ApJ...716.1573J. doi:10.1088/0004-637X/716/2/1573.
^ Zendejas, J.; Segura, A.; Raga, A.C. (2010). "Atmospheric mass loss by stellar wind from planets around main sequence M stars". Icarus. 210 (2): 539–544. arXiv:1006.0021. Bibcode:2010Icar..210..539Z. doi:10.1016/j.icarus.2010.07.013.
^ Masuda, K. (2014). "Very Low Density Planets Around Kepler-51 Revealed with Transit Timing Variations and an Anomaly Similar to a Planet-Planet Eclipse Event". The Astrophysical Journal. 783: 53. arXiv:1401.2885. Bibcode:2014ApJ...783...53M. doi:10.1088/0004-637X/783/1/53.
^ "Artist's impression of exoplanet orbiting two stars". www.spacetelescope.org. Retrieved 24 September 2016.
^ Petigura, E. A.; Howard, A. W.; Marcy, G. W. (2013). "Prevalence of Earth-size planets orbiting Sun-like stars". Proceedings of the National Academy of Sciences. 110 (48): 19273–19278. arXiv:1311.6806. Bibcode:2013PNAS..11019273P. doi:10.1073/pnas.1319909110. PMC 3845182
. PMID 24191033.
^ Cumming, Andrew; Butler, R. Paul; Marcy, Geoffrey W.; Vogt, Steven S.; Wright, Jason T.; Fischer, Debra A. (2008). "The Keck Planet Search: Detectability and the Minimum Mass and Orbital Period Distribution of Extrasolar Planets". Publications of the Astronomical Society of the Pacific. 120 (867): 531–554. arXiv:0803.3357. Bibcode:2008PASP..120..531C. doi:10.1086/588487.
^ Bonfils, X.; Forveille, T.; Delfosse, X.; Udry, S.; Mayor, M.; Perrier, C.; Bouchy, F.; Pepe, F.; Queloz, D.; Bertaux, J. -L. (2005). "The HARPS search for southern extra-solar planets". Astronomy and Astrophysics. 443 (3): L15–L18. arXiv:astro-ph/0509211. Bibcode:2005A&A...443L..15B. doi:10.1051/0004-6361:200500193.
^ Wang, J.; Fischer, D. A. (2014). "Revealing a Universal Planet–Metallicity Correlation for Planets of Different Solar-Type Stars". The Astronomical Journal. 149: 14. arXiv:1310.7830. Bibcode:2015AJ....149...14W. doi:10.1088/0004-6256/149/1/14.
^ Schwarz, Richard. Binary Catalogue of Exoplanets. Universität Wien
^ Schwarz, Richard. STAR-DATA. Universität Wien
^ NASA Hubble Finds a True Blue Planet. NASA. 11 July 2013
^ Evans, T. M.; Pont, F. D. R.; Sing, D. K.; Aigrain, S.; Barstow, J. K.; Désert, J. M.; Gibson, N.; Heng, K.; Knutson, H. A.; Lecavelier Des Etangs, A. (2013). "The Deep Blue Color of HD189733b: Albedo Measurements with Hubble Space Telescope/Space Telescope Imaging Spectrograph at Visible Wavelengths" (Full text). The Astrophysical Journal. 772 (2): L16. arXiv:1307.3239. Bibcode:2013ApJ...772L..16E. doi:10.1088/2041-8205/772/2/L16.
^ Kuzuhara, M.; Tamura, M.; Kudo, T.; Janson, M.; Kandori, R.; Brandt, T. D.; Thalmann, C.; Spiegel, D.; Biller, B.; Carson, J.; Hori, Y.; Suzuki, R.; Burrows, A.; Henning, T.; Turner, E. L.; McElwain, M. W.; Moro-Martín, A.; Suenaga, T.; Takahashi, Y. H.; Kwon, J.; Lucas, P.; Abe, L.; Brandner, W.; Egner, S.; Feldt, M.; Fujiwara, H.; Goto, M.; Grady, C. A.; Guyon, O.; Hashimoto, J.; et al. (2013). "Direct Imaging of a Cold Jovian Exoplanet in Orbit around the Sun-like Star GJ 504" (Full text). The Astrophysical Journal. 774 (11): 11. arXiv:1307.2886. Bibcode:2013ApJ...774...11K. doi:10.1088/0004-637X/774/1/11.
^ Carson; Thalmann; Janson; Kozakis; Bonnefoy; Biller; Schlieder; Currie; McElwain (15 November 2012). "Direct Imaging Discovery of a 'Super-Jupiter' Around the late B-Type Star Kappa And". The Astrophysical Journal. 763 (2): L32. arXiv:1211.3744[astro-ph.SR]. Bibcode:2013ApJ...763L..32C. doi:10.1088/2041-8205/763/2/L32.
^ The Apparent Brightness and Size of Exoplanets and their Stars, Abel Mendez, updated 30 June 2012, 12:10 PM
^ "Coal-Black Alien Planet Is Darkest Ever Seen". Space.com. Retrieved 12 August 2011.
^ Kipping, David M.; Spiegel, David S. (2011). "Detection of visible light from the darkest world". Monthly Notices of the Royal Astronomical Society: Letters. 417: L88–L92. arXiv:1108.2297. Bibcode:2011MNRAS.417L..88K. doi:10.1111/j.1745-3933.2011.01127.x.
^ Barclay, T.; Huber, D.; Rowe, J. F.; Fortney, J. J.; Morley, C. V.; Quintana, E. V.; Fabrycky, D. C.; Barentsen, G.; Bloemen, S.; Christiansen, J. L.; Demory, B. O.; Fulton, B. J.; Jenkins, J. M.; Mullally, F.; Ragozzine, D.; Seader, S. E.; Shporer, A.; Tenenbaum, P.; Thompson, S. E. (2012). "Photometrically derived masses and radii of the planet and star in the TrES-2 system". The Astrophysical Journal. 761: 53. arXiv:1210.4592. Bibcode:2012ApJ...761...53B. doi:10.1088/0004-637X/761/1/53.
^ abc Burrows, Adam (2014). "Scientific Return of Coronagraphic Exoplanet Imaging and Spectroscopy Using WFIRST". arXiv:1412.6097[astro-ph.EP].
^ Unlocking the Secrets of an Alien World's Magnetic Field, Space.com, by Charles Q. Choi, 20 November 2014
^ Kislyakova, K. G.; Holmstrom, M.; Lammer, H.; Odert, P.; Khodachenko, M. L. (2014). "Magnetic moment and plasma environment of HD 209458b as determined from Ly observations". Science. 346 (6212): 981–4. arXiv:1411.6875. Bibcode:2014Sci...346..981K. doi:10.1126/science.1257829. PMID 25414310.
^ Footprint of a Magnetic Exoplanet, www.skyandtelescope.com, 9 January 2004, Robert Naeye
^ Nichols, J. D. (2011). "Magnetosphere-ionosphere coupling at Jupiter-like exoplanets with internal plasma sources: Implications for detectability of auroral radio emissions". Monthly Notices of the Royal Astronomical Society. 414 (3): 2125–2138. arXiv:1102.2737. Bibcode:2011MNRAS.414.2125N. doi:10.1111/j.1365-2966.2011.18528.x.
^ Radio Telescopes Could Help Find Exoplanets. RedOrbit. 18 April 2011
^ "Radio Detection of Extrasolar Planets: Present and Future Prospects" (PDF). NRL, NASA/GSFC, NRAO, Observatoìre de Paris. Retrieved 15 October 2008.
^ Kean, Sam (2016). "Forbidden plants, forbidden chemistry". Distillations. 2 (2): 5. Retrieved 22 March 2018.
^ Super-Earths Get Magnetic 'Shield' from Liquid Metal, Charles Q. Choi, SPACE.com, 22 November 2012.
^ Buzasi, D. (2013). "Stellar Magnetic Fields As a Heating Source for Extrasolar Giant Planets". The Astrophysical Journal. 765 (2): L25. arXiv:1302.1466. Bibcode:2013ApJ...765L..25B. doi:10.1088/2041-8205/765/2/L25.
^ Chang, Kenneth (16 August 2018). "Settling Arguments About Hydrogen With 168 Giant Lasers - Scientists at Lawrence Livermore National Laboratory said they were "converging on the truth" in an experiment to understand hydrogen in its liquid metallic state". The New York Times. Retrieved 18 August 2018.
^ Staff (16 August 2018). "Under pressure, hydrogen offers a reflection of giant planet interiors - Hydrogen is the most-abundant element in the universe and the simplest, but that simplicity is deceptive". Science Daily. Retrieved 18 August 2018.
^ Valencia, Diana; O'Connell, Richard J. (2009). "Convection scaling and subduction on Earth and super-Earths". Earth and Planetary Science Letters. 286 (3–4): 492–502. Bibcode:2009E&PSL.286..492V. doi:10.1016/j.epsl.2009.07.015.
^ Van Heck, H.J.; Tackley, P.J. (2011). "Plate tectonics on super-Earths: Equally or more likely than on Earth". Earth and Planetary Science Letters. 310 (3–4): 252–261. Bibcode:2011E&PSL.310..252V. doi:10.1016/j.epsl.2011.07.029.
^ O'Neill, C.; Lenardic, A. (2007). "Geological consequences of super-sized Earths". Geophysical Research Letters. 34 (19): L19204. Bibcode:2007GeoRL..3419204O. doi:10.1029/2007GL030598.
^ Valencia, Diana; O'Connell, Richard J.; Sasselov, Dimitar D (November 2007). "Inevitability of Plate Tectonics on Super-Earths". Astrophysical Journal Letters. 670 (1): L45–L48. arXiv:0710.0699. Bibcode:2007ApJ...670L..45V. doi:10.1086/524012.
^ Super Earths Likely To Have Both Oceans and Continents, astrobiology.com. 7 January 2014
^ Cowan, N. B.; Abbot, D. S. (2014). "Water Cycling Between Ocean and Mantle: Super-Earths Need Not Be Waterworlds". The Astrophysical Journal. 781: 27. arXiv:1401.0720. Bibcode:2014ApJ...781...27C. doi:10.1088/0004-637X/781/1/27.
^ Michael D. Lemonick (6 May 2015). "Astronomers May Have Found Volcanoes 40 Light-Years From Earth". National Geographic. Retrieved 8 November 2015.
^ Demory, Brice-Olivier; Gillon, Michael; Madhusudhan, Nikku; Queloz, Didier (2015). "Variability in the super-Earth 55 Cnc e". Monthly Notices of the Royal Astronomical Society. 455 (2): 2018–2027. arXiv:1505.00269. Bibcode:2016MNRAS.455.2018D. doi:10.1093/mnras/stv2239.
^ Scientists Discover a Saturn-like Ring System Eclipsing a Sun-like Star, Space Daily, 13 January 2012
^ Mamajek, E. E.; Quillen, A. C.; Pecaut, M. J.; Moolekamp, F.; Scott, E. L.; Kenworthy, M. A.; Cameron, A. C.; Parley, N. R. (2012). "Planetary Construction Zones in Occultation: Discovery of an Extrasolar Ring System Transiting a Young Sun-Like Star and Future Prospects for Detecting Eclipses by Circumsecondary and Circumplanetary Disks". The Astronomical Journal. 143 (3): 72. arXiv:1108.4070. Bibcode:2012AJ....143...72M. doi:10.1088/0004-6256/143/3/72.
^ Kalas, P.; Graham, J. R.; Chiang, E.; Fitzgerald, M. P.; Clampin, M.; Kite, E. S.; Stapelfeldt, K.; Marois, C.; Krist, J. (2008). "Optical Images of an Exosolar Planet 25 Light-Years from Earth". Science. 322 (5906): 1345–8. arXiv:0811.1994. Bibcode:2008Sci...322.1345K. doi:10.1126/science.1166609. PMID 19008414.
^ Schlichting, Hilke E.; Chang, Philip (2011). "Warm Saturns: On the Nature of Rings around Extrasolar Planets That Reside inside the Ice Line". The Astrophysical Journal. 734 (2): 117. arXiv:1104.3863. Bibcode:2011ApJ...734..117S. doi:10.1088/0004-637X/734/2/117.
^ Bennett, D. P.; Batista, V.; Bond, I. A.; Bennett, C. S.; Suzuki, D.; Beaulieu, J. -P.; Udalski, A.; Donatowicz, J.; Bozza, V.; Abe, F.; Botzler, C. S.; Freeman, M.; Fukunaga, D.; Fukui, A.; Itow, Y.; Koshimoto, N.; Ling, C. H.; Masuda, K.; Matsubara, Y.; Muraki, Y.; Namba, S.; Ohnishi, K.; Rattenbury, N. J.; Saito, T.; Sullivan, D. J.; Sumi, T.; Sweatman, W. L.; Tristram, P. J.; Tsurumi, N.; Wada, K.; et al. (2014). "MOA-2011-BLG-262Lb: A sub-Earth-mass moon orbiting a gas giant or a high-velocity planetary system in the galactic bulge". The Astrophysical Journal. 785 (2): 155. arXiv:1312.3951. Bibcode:2014ApJ...785..155B. doi:10.1088/0004-637X/785/2/155.
^ "Cloudy versus clear atmospheres on two exoplanets". www.spacetelescope.org. Retrieved 6 June 2017.
^ Charbonneau, David; et al. (2002). "Detection of an Extrasolar Planet Atmosphere". The Astrophysical Journal. 568 (1): 377–384. arXiv:astro-ph/0111544. Bibcode:2002ApJ...568..377C. doi:10.1086/338770.
^ Evaporating exoplanet stirs up dust. Phys.org. 28 August 2012
^ Woollacott, Emma (18 May 2012) New-found exoplanet is evaporating away. TG Daily
^ Bhanoo, Sindya N. (25 June 2015). "A Planet with a Tail Nine Million Miles Long". The New York Times. Retrieved 25 June 2015.
^ St. Fleur, Nicholas (19 May 2017). "Spotting Mysterious Twinkles on Earth From a Million Miles Away". The New York Times. Retrieved 20 May 2017.
^ Marshak, Alexander; Várnai, Tamás; Kostinski, Alexander (15 May 2017). "Terrestrial glint seen from deep space: oriented ice crystals detected from the Lagrangian point". Geophysical Research Letters. 44 (10): 5197–5202. Bibcode:2017GeoRL..44.5197M. doi:10.1002/2017GL073248.
^ Forget "Earth-Like"—We'll First Find Aliens on Eyeball Planets, Nautilus, Posted by Sean Raymond on 20 February 2015
^ Dobrovolskis, Anthony R. (2015). "Insolation patterns on eccentric exoplanets". Icarus. 250: 395–399. Bibcode:2015Icar..250..395D. doi:10.1016/j.icarus.2014.12.017.
^ Tony Dobrovolskis (18 March 2014). "Patterns of Sunlight on Extra-Solar Planets". SETI Institute. Retrieved 25 March 2014.
^ "Oxygen Is Not Definitive Evidence of Life on Extrasolar Planets". NAOJ. Astrobiology Web. 10 September 2015. Retrieved 2015-09-11.
^ Kopparapu, Ravi Kumar (2013). "A revised estimate of the occurrence rate of terrestrial planets in the habitable zones around kepler m-dwarfs". The Astrophysical Journal Letters. 767 (1): L8. arXiv:1303.2649. Bibcode:2013ApJ...767L...8K. doi:10.1088/2041-8205/767/1/L8.
^ Cruz, Maria; Coontz, Robert (2013). "Exoplanets - Introduction to Special Issue". Science. 340 (6132): 565. doi:10.1126/science.340.6132.565. Retrieved 18 May 2013.
Further reading
Boss, Alan (2009). The Crowded Universe: The Search for Living Planets. Basic Books. ISBN 978-0-465-00936-7 (Hardback); ISBN 978-0-465-02039-3 (Paperback).
Dorminey, Bruce (2001). Distant Wanderers. Springer-Verlag. ISBN 978-0-387-95074-7 (Hardback); ISBN 978-1-4419-2872-6 (Paperback).
Jayawardhana, Ray (2011). Strange New Worlds: The Search for Alien Planets and Life beyond Our Solar System. Princeton, NJ: Princeton University Press. ISBN 978-0-691-14254-8 (Hardcover).
Perryman, Michael (2011). The Exoplanet Handbook. Cambridge University Press. ISBN 978-0-521-76559-6.
Seager, Sara, ed. (2011). Exoplanets. University of Arizona Press. ISBN 978-0-8165-2945-2.
Villard, Ray; Cook, Lynette R. (2005). Infinite Worlds: An Illustrated Voyage to Planets Beyond Our Sun. University of California Press. ISBN 978-0-520-23710-0.
Yaqoob, Tahir (2011). Exoplanets and Alien Solar Systems. New Earth Labs (Education and Outreach). ISBN 978-0-9741689-2-0 (Paperback).
van Dishoeck, Ewine F.; Bergin, Edwin A.; Lis, Dariusz C.; Lunine, Jonathan I. (2014). "Water: From Clouds to Planets". Protostars and Planets VI. arXiv:1401.8103[astro-ph.GA]. Bibcode:2014prpl.conf..835V. doi:10.2458/azu_uapress_9780816531240-ch036. ISBN 978-0-8165-3124-0.
External links
![]() | Wikimedia Commons has media related to Exoplanets. |
![]() | Wikiversity has learning resources about Observational astronomy/Extrasolar planet |
The Extrasolar Planets Encyclopaedia (Paris Observatory)- NASA Exoplanet Archive
- Open Exoplanet Catalogue
The Habitable Exoplanets Catalog (PHL/UPR Arecibo)
Extrasolar Planets – D. Montes, UCM
Exoplanets at Paris Observatory- Graphical Comparison of Extrasolar Planets